Abstract
Purpose
This study aims to identify the location of the micropyle, the role of the micropyle in seed germination and the association between the micropyle size and seed weight of grass peas.
Design/methodology/approach
First, the micropyle was identified by cutting the seed in half and observing the seeds under the electron microscope. Second, the micropyle was covered by lanolin to block water imbibition. The rate of imbibition and germination was then observed. Lastly, micropyle sizes of various grass pea genotypes were identified by capturing seed images under a light microscope and converting the sizes to mm2 using computer software (ImageJ).
Findings
The location of micropyle was located nearby the hilum, similar to soybean seeds. Seed imbibition was significantly lower in lanolin application (<87%) than in the control (>124%) after 24 hours of submergence. Germination was a day delay for lanolin application on the micropyle compared to lanolin application on the non-micropyle. The germination delay resulted in a significantly lower germination percentage at <57% on the micropyle lanolin application than at >79% on the non-micropyle lanolin application after 10 days of sowing. There is no correlation between the micropyle size and seed weight.
Originality/value
These findings add information on the location and the role of the micropyle for grass pea seed germination.
Keywords
Citation
Wiraguna, E. (2024), "The role of micropyle for grass pea germination", Arab Gulf Journal of Scientific Research, Vol. 42 No. 3, pp. 722-729. https://doi.org/10.1108/AGJSR-01-2023-0015
Publisher
:Emerald Publishing Limited
Copyright © 2023, Edi Wiraguna
License
Published in Arab Gulf Journal of Scientific Research. Published by Emerald Publishing Limited. This article is published under the Creative Commons Attribution (CC BY 4.0) licence. Anyone may reproduce, distribute, translate and create derivative works of this article (for both commercial and non-commercial purposes), subject to full attribution to the original publication and authors. The full terms of this licence may be seen at http://creativecommons.org/licences/by/4.0/legalcode
Introduction
Grass pea (Lathyrus sativus L.) is one of the important legume crops in Bangladesh, Ethiopia and Pakistan (Benková & Žáková, 2001; Campbell et al., 1994; Tadesse & Bekele, 2001; Yigzaw et al., 2001). Grass pea contains high protein in seeds higher than chickpea (Cicer arietinum L., ∼18%) and similar to that of field pea (Pisum sativum L.) and faba bean (Vicia faba L.) (Yan et al., 2006). Grass pea is also reputed to be tolerant to abiotic stresses such as flooding and low soil fertility (Benková & Žáková, 2001; Campbell et al., 1994; Tadesse & Bekele, 2001; Yigzaw et al., 2001).
Solute imbibed into seeds (imbibition) is through the micropyle and/or seed coat (Manohar & Heydecker, 1964; Edelstein, Corbineau, Kigel, & Nerson, 1995; Munz et al., 2017). In seeds of Cucumis melo (melon), solute imbibes into the seeds through the micropyle, and the seed coat shown by intercellular spaces on the outer layer of the seed coat (Edelstein et al., 1995). However, when there are no intercellular spaces in the outer layer of the seed coat, the imbibition is only through micropyle as shown in seeds of Geranium carolinianum (Gama-Arachchige, Baskin, Geneve, & Baskin, 2011). However, the information on the imbibition pathway of grass peas is still unknown.
A micropyle is a small pore in the seed coat of ripe seeds that allows water and oxygen to enter during germination (Manohar & Heydecker, 1964; Munz et al., 2017). In Rhus sp. and G. carolinianum, the micropyle is located at the tip of the seed nearby the hilum and acts as the primary site of water absorption (Li, Baskin, & Baskin, 1999; Gama-Arachchige, Baskin, Geneve, & Baskin, 2010; Munz et al., 2017). The germination process in seeds begins with the absorption of water by the micropyle, which leads to the activation of enzymes that break down the stored nutrients and release the energy necessary for the growth of the embryonic axis (Bewley, 1997; Munz et al., 2017). Therefore, the micropyle is a critical structure for successful germination and early seedling growth. However, there is no information on the micropyle location of grass pea seeds.
The rate of seed imbibition is associated with waterlogging tolerance (Tian, Nakamura, & Kokubun, 2005; Zhang, Chen, Chen, Wang, & Li, 2008), and seed imbibition is related to micropyle (Manohar & Heydecker, 1964). Oxygen dissolved in water or solution that imbibes through the micropyle and/or seed coat into an embryo is used to produce energy for germination (Chen, 1988; Couee, Defontaine, Carde, & Pradet, 1992; Bewley, 1997; Budko et al., 2013). If there is insufficient oxygen, germination may fail (Al-Ani et al., 1985) due to low energy production in the embryo (Bewley, 1997; Narsai, Rocha, Geigenberger, Whelan, & Van Dongen, 2011). In addition, waterlogging tolerance in grass peas is related to seed size (Wiraguna, Malik, Colmer, & Erskine, 2020). However, there is no information on a correlation between micropyle size and waterlogging tolerance.
Lanolin is used to reduce solute imbibition and oxygen infiltration during germination and root growth (Edelstein et al., 1995; Pimentel et al., 2014; Alves Junior, de Oliveira Vitoriano, da Silva, de Lima Farias, & de Lima Dantas, 2016; Ejiri & Shiono, 2019). Edelstein et al. (1995) added that covering lanolin on the seed micropyle delays germination for two days and reduces germination percentage from 100% to 50% after 7 days in temperature-controlled germination chambers. Moreover, covering the melon seed micropyle with lanolin and placing it at a low temperature (15 °C) inhibits the melon seeds from germination (Edelstein et al., 1995).
This study was designed to investigate three hypotheses: (1) the micropyle of grass pea seeds is located nearby the hilum, (2) water imbibes into grass pea seeds through the micropyle, and (3) there is a correlation between the micropyle size and the seed weight of grass pea seeds.
Materials and methods
The study comprised three experiments that was designed to test the role of the micropyle during seed imbibition on grass pea seeds. Exp. 1 was to identify a location of the micropyle, Exp. 2 was to investigate water penetration during imbibition by covering the micropyle with lanolin (Edelstein et al., 1995; Alves Junior et al., 2016), and Exp. 3 was to test an association between the seed weight and micropyle size. These experiments were carried out using an electron microscope in CMCA (Centre for Microscopy, Characterisation and Analysis) for Exp. 1 and in a controlled temperature room (25 °C) for Exp. 2 and 3, University of Western Australia, Perth.
Exp. 1 seed observation
The Exp. 1 was carried out to identify the location of the micropyle and other seed tissues. A dry seed of grass pea genotype Ceora were cut longitudinally into half and placed on a cryostage in a JOEL JCM-7000 scanning electron microscope for observation for ∼30 min with the cross-section facing the sensor of the electron microscope. Identification of the micropyle, hilum and radicle was then carried out.
Exp. 2 water uptake during seed imbibition
The same grass pea genotype as Exp. 1 was tested in Exp. 2 to identify the role of the micropyle during seed imbibition and germination. The factor of Exp. 2 was the positions of the lanolin on the micropyle (one dot), on the opposite side of the micropyle (one dot), on the micropyle and the opposite side of the micropyle (two dots) and the control (without covering the seed coats). A complete randomised design was applied with three replicates in this experiment. This experiment was conducted in the 25 °C control temperature room in the dark.
A 10 L solution of 0.5 mM CaSO4 was prepared one day before the experiment and placed in the control room temperature (25 °C). Approximately 1 g of grass pea seed (genotype Ceora) per treatment per replicate was submerged in a 250 mL flask containing 100 mL of the prepared solution for 24 h. Seed weight increment was measured as a percentage of seed weight increase (Zhang et al., 2008).
Two layers of saturated filter papers (Whatman no. 50) were placed on Petri dishes (90 mm diameter). Ten seeds were then put on the saturated filter paper. The seeds were covered with a layer of filter paper and kept in a controlled temperature room at 25 °C in the dark for 10 days (Kranner, Roach, Beckett, Whitaker, & Minibayeva, 2010; Wiraguna, Malik, & Erskine, 2017, 2020, 2021). Seeds were categorised as germination when the radicles emerged for more than 5 mm in length. There were three replicates for each treatment and observation was carried out daily.
Exp. 3 association between seed weight and micropyle size of grass pea
Nine grass pea genotypes from different countries of origins were selected to identify a relation between the seed weight and micropyle size (Table 1). The weight of one hundred seeds was recorded after threshing the grass pea pods as described by Wiraguna et al. (2017, 2020). Micropyle areas were measured after collecting photographs of the seed micropyle under a light microscope with 20× magnificent. The micropyle photograph was then analysed using computer software (ImageJ) to measure micropyle areas as described by Abràmoff, Magalhães, and Ram (2004).
Data analysis
The data were analysed by analysis of variance (ANOVA) to test the effect of location on seed coat in Exp. 2 and by Pearson correlation to test the association between traits in Exp. 3 using R-studio (2022.12.0 + 353) and GenStat 20th edition (VSN International, UK). Mean differences were indicated by the least significant difference (LSD) at p = 0.05.
Results
Exp. 1 grass pea seed observation
Micropyle showed as a small tunnel connected between inside and outside seeds. The location of the micropyle was nearby a radicle (Figure 1). The micropyle was located on the end of the hilum.
Exp. 2 seed water uptake during imbibition
A one-way ANOVA showed the location of lanolin on the seed coat was significant (p < 0.05). The application of lanolin on micropyle significantly reduced percent seed weight increment by 36.6% relative to the control (Figure 2). The seed weight increment between treatments of lanolin application was not significantly different and ranged between 80 and 90% (Figure 2).
The treatments of lanolin significantly reduced germination percentage (p < 0.05). Control and lanolin placed on the seed coat started germination on the first day of treatment, but germination was delayed for a day for the treatment of lanolin placed on the micropyle (Figure 3). Moreover, germination was significantly lower at <57% for the seeds covered by lanolin on the micropyle than the control at 87%.
Exp. 3 association between seed weight and micropyle size of grass pea
There was no significant correlation between the seed weight and the micropyle size (Figure 4). Therefore, waterlogging tolerance was not associated with micropyle size.
Discussion
Imbibition from the environment to seed embryos has been suggested mainly through the micropyle (Manohar & Heydecker, 1964; Munz et al., 2017). However, there is limited information on the micropyle and the role of the micropyle in seed germination. Moreover, a relation between the seed weight and the micropyle size on grass pea seeds is unknown. In this study, it was found that the location of grass pea micropyle nearby the hilum is similar to that shown in seeds of G. carolinianum (Gama-Arachchige et al., 2011) and Rhus sp. (Li, Baskin, & Baskin, 1999). The imbibition rate was lower in seed coat and/or micropyle closure than in the control, but there was no difference on seed imbibition rate between treatments (Figure 2). The micropyle closure delayed and significantly reduced the percent germination (Figure 3). This finding showed that the delay of imbibition resulted in the failure of germination by 30% similar to that shown for field peas (Manohar & Heydecker, 1964; Larson, 1968; Edelstein et al., 1995). The correlation between the seed weight and the size of the micropyle among grass pea genotypes was insignificant (Figure 3).
The location of the micropyle on the seed coat has been found nearby the hilum (Figure 1). This finding is similar to that shown for seeds of Picea abies (L.) Karst (Tillman-Sutela & Kauppi, 1995), G. carolinianum (Gama-Arachchige et al., 2010) and soybean (Muramatsu et al., 2008).
Imbibition from the environment (outside the seed) to the embryo was suggested mainly through micropyle (Manohar & Heydecker, 1964; Munz et al., 2017). The rate of seed imbibition was similar between treatments but lower than the control (Tillman-Sutela & Kauppi, 1995; Edelstein et al., 1995) (Figure 2). The treatment of covering micropyle significantly reduced percent germination (Figure 3). Percent germination was slower for micropyle seeds covered by lanolin than those uncovered by lanolin (Figure 3). This finding suggested that the micropyle plays a key role during the germination of grass pea seeds, similar to seeds of field peas, where the field pea seeds failed to germinate or reduced percent germination for the treatment of micropyle closure (Manohar & Heydecker, 1964).
Micropyle size was not associated with seed weight and waterlogging tolerance in grass peas (Table 1; Figure 4), but both traits were probably related to hilum size (Muramatsu et al., 2008). In soybean, for example, small seeds with a large proportion of hilum were more tolerant to waterlogging than large seeds with a large proportion of hilum, presumably, because hilum was used as a reservoir to keep moisture for seeds to germinate and survive during drainage after waterlogging (Tian et al., 2005; Muramatsu et al., 2008).
Conclusion
Micropyle and seed coat are pathways of water or solution to enter the seed and reach the grass pea seed embryo. The location of the micropyle nearby the hilum is similar to other seeds such as soybean and G. carolinianum. The rate of seed imbibition for 24 hours was lower than the control, but the rate of seed imbibition between treatments was similar. Percent germination was significantly reduced by covering the micropyle with lanolin. The lanolin application on micropyle also delayed germination by a day compared to the control. There was no association between micropyle size and seed weight.
Figures
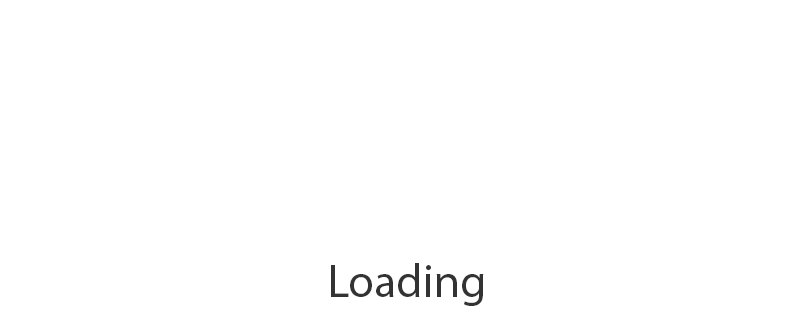
Figure 3
Germination percentage of grass pea seeds with treatments of lanolin placed on micropyle with a square symbol (■), on opposite site of micropyle (seed coat) with a triangle symbol (▲), on micropyle and opposite site of micropyle (seed coat) with a parallelogram symbol (♦) and control with a circle symbol (●)
Name of grass pea genotypes from different country origin
No. | Name of genotypes | Origin | 100 seed weight (g) | Response to soil waterlogging |
---|---|---|---|---|
1 | 8604 | Bangladesh | 9.5e ± 0.1 | Tolerant |
2 | Ceora | Australia | 14.3c ±0.3 | Sensitive |
3 | CPI 20495 | Cyprus | 22.4a ± 0.1 | Sensitive |
4 | CPI 9997 | Cyprus | 15.6b ± 0.1 | Sensitive |
5 | GP. 13 | Ethiopia | 11.9d ± 0.5 | Tolerant |
6 | GP. 29 | Ethiopia | 9.0e ± 0.1 | Tolerant |
7 | IFLA 251 | Afghanistan | 15.5b ± 0.2 | Sensitive |
8 | K 209.12 | Pakistan | 6.3f ± 0.1 | Sensitive |
9 | Site 41.4 | Greece | 8.7e ± 0.2 | Sensitive |
Note(s): Means are followed by standard error (n = 3). Differences in 100 seed weight between genotypes are shown as different letters (p < 0.001). Response to soil waterlogging on each genotype referred to Wiraguna et al. (2020)
References
Abràmoff, M. D., Magalhães, P. J., & Ram, S. J. (2004). Image processing with ImageJ. Biophotonics International, 11(7), 36–42.
Al-Ani, A., Bruzau, F., Raymond, P., Saint-Ges, V., Leblanc, J. M., & Pradet, A. (1985). Germination, respiration, and adenylate energy charge of seeds at various oxygen partial pressures. Plant Physiology, 79, 885–890.
Alves Junior, C., de Oliveira Vitoriano, J., da Silva, D. L. S., de Lima Farias, M., & de Lima Dantas, N. B. (2016). Water uptake mechanism and germination of Erythrina velutina seeds treated with atmospheric plasma. Scientific Reports, 6(1), 1–7.
Benková, M., & Žáková, M. (2001). Evaluation of selected traits in grasspea (Lathyrus sativus L.) genetic resources. Lathyrus Lathyrism Newsletter, 2, 27–30.
Bewley, J. D. (1997). Seed germination and dormancy. The Plant Cell, 9, 1055–1066.
Budko, N., Corbetta, A., Van Duijn, B., Hille, S., Rottschäfer, V., Wiegman, L. & Zhelyazov, D. (2013) Oxygen transport and consumption in germinating seeds. In: Heydenreich, M. O., Hille, S.C., Rottschäfer, V., Spieksma, F., & Verbitskiy, E. (eds.), Proceedings of the 90th European Study Group Mathematics in Industry (SWI 2013, Leiden, The Netherlands, January 28-February 1, 2013) (pp 5-30). Leiden: Lorentz Center.
Campbell, C., Mehra, R., Agrawal, S., Chen, Y., Moneim, A.A., Khawaja, H., Yadov, C., Tay, J., & Araya, W. (1994). Current status and future strategy in breeding grass pea (Lathyrus sativus). Euphytica, 73, 167–175.
Chen, L. B. (1988). Mitochondrial membrane potential in living cells. Annual Review Cell Biology, 4, 155–181.
Couee, I., Defontaine, S., Carde, J. P., & Pradet, A. (1992). Effects of anoxia on mitochondrial biogenesis in rice shoots: Modification of in Organello translation characteristics. Plant Physiology, 98, 411–421.
Edelstein, M., Corbineau, F., Kigel, J., & Nerson, H. (1995). Seed coat structure and oxygen availability control low temperature germination of melon (Cucumis melo) seeds. Physiologia Plantarum, 93(3), 451–456.
Ejiri, M., & Shiono, K. (2019). Prevention of radial oxygen loss is associated with exodermal suberin along adventitious roots of annual wild species of Echinochloa. Frontiers in Plant Science, 10, 1–13.
Gama-Arachchige, N., Baskin, J., Geneve, R., & Baskin, C. (2010). Identification and characterization of the water gap in physically dormant seeds of Geraniaceae, with special reference to Geranium carolinianum. Annals of Botany, 105(6), 977–990.
Gama-Arachchige, N. S., Baskin, J. M., Geneve, R. L., & Baskin, C. C. (2011). Acquisition of physical dormancy and ontogeny of the micropyle–water-gap complex in developing seeds of Geranium carolinianum (Geraniaceae). Annals of Botany, 108(1), 51–64.
Kranner, I., Roach, T., Beckett, R. P., Whitaker, C., & Minibayeva, F. V. (2010). Extracellular production of reactive oxygen species during seed germination and early seedling growth in Pisum sativum. Journal of Plant Physiology, 167(10), 805–811.
Larson, L. (1968). The effect soaking pea seeds with or without seedcoats has on seedling growth. Plant Physiology, 43(2), 255–259.
Li, X., Baskin, J. M., & Baskin, C. C. (1999). Anatomy of two mechanisms of breaking physical dormancy by experimental treatments in seeds of two North American Rhus species (Anacardiaceae). American Journal of Botany, 86, 1505–1511.
Manohar, M. S., & Heydecker, W. (1964). Effects of water potential on germination of pea seeds. Nature, 202, 22–24.
Munz, E., Rolletschek, H., Oeltze‐Jafra, S., Fuchs, J., Guendel, A., Neuberger, T., … Borisjuk, L. (2017). A functional imaging study of germinating oilseed rape seed. New Phytologist, 216(4), 1181–1190.
Muramatsu, N., Kokubun, M., & Horigane, A. (2008). Relation of seed structures to soybean cultivar difference in pre-germination flooding tolerance. Plant Production Science, 11, 434–439.
Narsai, R., Rocha, M., Geigenberger, P., Whelan, J., & Van Dongen, J. T. (2011). Comparative analysis between plant species of transcriptional and metabolic responses to hypoxia. New Phytologist, 190, 472–487.
Pimentel, P., Almada, R. D., Salvatierra, A., Toro, G., Arismendi, M. J., Pino, M. T., … Pinto, M. (2014). Physiological and morphological responses of Prunus species with different degree of tolerance to long-term root hypoxia. Scientia Horticulturae, 180, 14–23.
Tadesse, W., & Bekele, E. (2001). Isozymes variability of grasspea (Lathyrus sativus L.) in Ethiopia. Lathyrus Lathyrism Newsletter, 2, 43–46.
Tian, X. -H., Nakamura, T., & Kokubun, M. (2005). The role of seed structure and oxygen responsiveness in pre-germination flooding tolerance of soybean cultivars. Plant Production Science, 8(2), 157–165.
Wiraguna, E., Malik, A. I., & Erskine, W. (2017). Waterlogging tolerance in lentil (Lens culinaris Medik. subsp. culinaris) germplasm associated with geographic origin. Genetic Resources and Crop Evolution, 64, 579–586.
Tillman-Sutela, E., & Kauppi, A. (1995). The significance of structure for imbibition in seeds of the Norway spruce, Picea abies (L.) Karst. Trees, 9, 269–278.
Wiraguna, E., Malik, A. I., Colmer, T. D., & Erskine, W. (2020). Waterlogging tolerance of grass pea (Lathyrus sativus L.) at germination related to country of origin. Experimental Agriculture, 56(6), 837–850.
Wiraguna, E., Malik, A. I., Colmer, T. D., & Erskine, W. (2021). Tolerance of four grain legume species to waterlogging, hypoxia and anoxia at germination and recovery. AoB Plants, 13(4), 1–13.
Yan, Z.Y., Spencer, P.S., Li, Z.X., Liang, Y.M., Wang, Y.F., Wang, C.Y., & Li, F.M. (2006). Lathyrus sativus (grass pea) and its neurotoxin ODAP. Phytochemistry, 67, 107–121.
Yigzaw, Y., Gorton, L., Akalu, G., & Solomon, T. (2001). Fermentation of teff (Eragrostis tef), grass-pea (Lathyrus sativus), and their mixtures: aspects of nutrition and food safety. Lathyrus Lathyrism Newsletter, 2, 8–10.
Zhang, X., Chen, J., Chen, L., Wang, H., & Li, J. (2008). Imbibition behavior and flooding tolerance of rapeseed seed (Brassica napus L.) with different testa color. Genetic Resources and Crop Evolution, 55, 1175–1184.
Further reading
Campbell, C. G. (1997). Grass pea, Lathyrus sativus L, Promoting the conservation and use of underutilized and neglected crops (Vol. 18, pp. 8–53). Rome: Institute of Plant Genetics and Crop Plant Research, Gatersleben/International Plant Genetic Resources Institute.
Acknowledgements
Author thanks the Indonesia Endowment Fund for Education, the Ministry of Finance of the Republic of Indonesia (LPDP) and College of Vocational Studies, IPB University. The author is thankful to William Erskine, Timothy Colmer, Imran Malik and Peta Clode for advice on data collection and analysis.